CMS Confirms a Rare Phenomenon: Light Bouncing off Light
6 January 2025
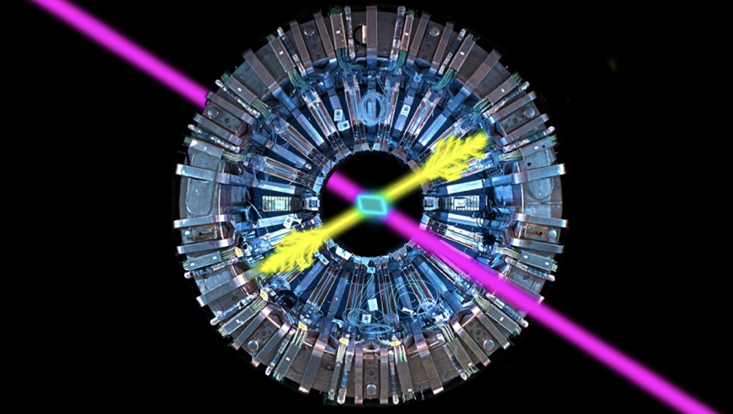
Photo: CMS Collaboration
Light particles bouncing off other light particles have been observed by the CMS collaboration at the LHC, confirming a rare prediction of the quantum nature of electromagnetism. Jeremi Niedziela (DESY) and Freya Blekman (DESY and UHH) of the Cluster of Excellence Quantum Universe led this analysis which also probed axion-like particles in new ranges.
According to quantum physics, light can behave like particles, called photons, under the right circumstances. The quantum description of light also predicts that it is impossible for photons to bounce off each other directly, but, in rare cases, it could look like they do by creating intermediate particles and finally returning to photons while conserving all the energy of the original photons. These extremely rare elastic light collisions were already predicted by the founders of quantum physics, like Enrico Fermi, and further worked on when Richard Feynman and many others developed the description of particle behavior for electromagnetism that eventually led to the standard model of particle physics we know and love today.
Physicists call these extremely rare collisions light-by-light scattering. Finding and studying these elastic light collisions is interesting because it is the most direct way to test the physics of electromagnetism at the LHC’s very high energies.
Many extremely rare things must happen simultaneously to scatter light from light. When charged particles move fast in an accelerator like the LHC, they can emit photons, which is rare. The emission of these photons is millions of times more frequent for lead ions than for protons, making it worthwhile to use the LHC lead ion runs to search for light-by-light scattering. Having those photon emissions happen with two lead ions close to each other is again very rare, and finally, the chance that the two photons bounce off each other is also extremely small.
To complicate things even further, many other collisions can look similar, resulting in two photons observed in the detector but coming from processes other than light-by-light scattering. Fortunately, electromagnetism is a very precise physics theory, and we have calculated that from the angles between the photons in the CMS experiment, it is possible to disentangle different kinds of LHC photon collisions and isolate light-by-light scattering.
Learning how to isolate the light-by-light scattering collisions also had a great byproduct. With the same technique and only minor changes, the creation of electrons from light can also be observed. This is a thousand times more likely and is called the Breit–Wheeler process. Studying these collisions gives another very precise way to test quantum electrodynamics, confirming that the measurement is consistent with the Standard Model prediction.
In a recent paper, CMS physicists have isolated 26 LHC collisions that are consistent with light-by-light scattering. In comparison, there should only be 12 background collisions out of the billions and billions of LHC collisions. The chance that the 14 extra collisions are all background is extremely small, less than 1 in 10 million, and above the standard particle physics threshold for the discovery of five standard deviations. The ATLAS collaboration also observed light-by-light scattering a few years ago, and CMS now confirms that this process is indeed possible at colliders. Because the calorimeter of the CMS experiment is particularly good at seeing photons at low energies, this measurement is complementary and gives more information for those collisions.
As a bonus, even with the modest number of light-by-light scattering candidates, we can search for undiscovered particles that interact (almost) only with photons - the observed number gives information on which particles can exist. This is the case for gravitons but also axion-like particles (ALPs), which would mediate interactions between the Standard Model and dark particles. Thanks to the light-by-light analysis, CMS is now sensitive to their properties that have not been accessible by any previous experiment.
Currently, the number of light-by-light scattering collisions is still very small. This means larger datasets could help CMS physicists study this intriguing electromagnetic phenomenon better because we will have more collisions to study. Furthermore, given the fact that we only observe 26 collisions in the signal region, each marginal enhancement plays a crucial role in refining our analyses. However, to be successful, it is also essential to have a really good detector, as the busy LHC collisions will eventually affect the CMS electromagnetic calorimeter to the point that this measurement would be difficult. This will affect many CMS analyses, including the study of the Higgs boson, and a new High Granularity Calorimeter promises to improve the performance of the CMS detector during High Luminosity LHC.