Riding the Dark Matter Wave
19 March 2024
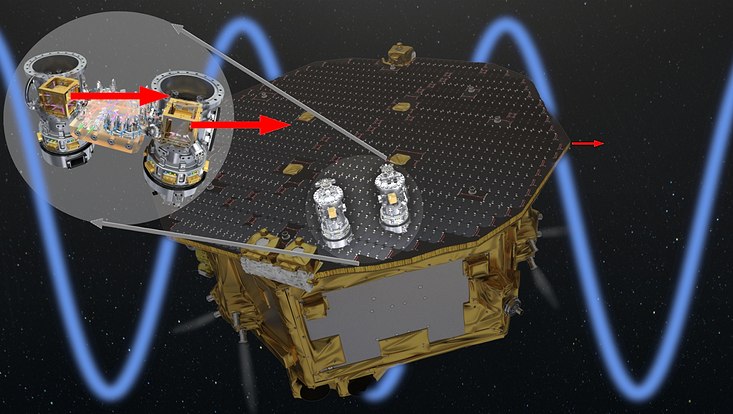
Photo: J Frerick / ESA / ATG medialab.
Dark Matter, the elusive particle that has captivated theorists and experimentalists alike, continues to evade direct observation, necessitating innovative strategies to match the advancement of Dark Matter detection techniques. Recently, Jonas Frerick (DESY), Jörg Jäckel (University of Heidelberg), Felix Kahlhöfer (Karlsruhe Institute of Technology) and Kai Schmidt-Hoberg (DESY) have unveiled a new approach to constrain a broad class of Dark Matter models. The group of theorists has studied the potential use of the LISA Pathfinder satellite as a dark matter direct detection experiment. This satellite flew in 2015‒2017 as a precursor to the forthcoming LISA mission — a space-based gravitational wave detector.
Cosmology tells us that Dark Matter is all around but according to our best estimates, the local density of Dark Matter is less than a proton mass per cubic centimeter! This is much less than the best human-made vacuum. But as long as the Dark Matter particles are heavy, with mass roughly between a proton and the heaviest atoms, we have good chances to detect them. Living in this sea of Dark Matter particles implies that we are constantly bombarded by these particles. That is why physicists build very well-shielded and ultra-sensitive experiments to look for collisions between the Dark Matter particles and the nuclei of the atoms.
Unfortunately, these experiments have not observed such interactions yet. Therefore, a part of the community became more interested in much lighter (meaning much lighter than protons) Dark Matter candidates that, on top of being less massive, also interact even more weakly with the surrounding particles that we know and love. This is a real issue because the lighter the particle, the smaller the effect on an atom in a collision. Playing pool is a good analogy: as long as the cue ball is similar to the mass of the other balls, the effect of a collision will be sizeable. Now imagine you have to play but someone replaces the cue ball by a grain of sand. Good luck moving the other balls…
Let us focus on a particular type of ultra-light Dark Matter candidate. This specific type is a so-called Dark Photon, a twin brother of our well-known photon, the particle of light. In this context, “dark” refers to the particle interacting very weakly and thus being essentially invisible. It doesn’t mean that the Dark Photon is very good at absorbing light or appears dark in the day-to-day sense. Due to the low mass, the Dark Photon Dark Matter particles conspire to form a dark electric field which surrounds us but it never shows up in everyday life due to its weak interactions.
In some models which are especially interesting from a theoretical perspective, this dark electric field has very fascinating properties due to its specific type of couplings. The standard electric field couples to electric charges and accelerates them. However, this dark electric field preferably couples to the Baryon-Lepton number, which is the difference between the number of baryons (the family of particles including protons and neutrons) and the number of leptons (the family of particles including electrons). We know that the number of protons and electrons must be equal in an atom due to electric charge neutrality. This means that the Baryon-Lepton number is just given by the number of neutrons inside the atom. In conclusion, because atoms have neutrons, they are not ”neutral” for the dark electric field and can be accelerated by this type of dark matter!
We can now plan a very simple direct detection experiment. We just put a probe, preferably made from atoms with a lot of neutrons, in a very well-shielded environment and look for a tiny acceleration from the Dark Photon Dark Matter-induced dark electric field. This is where the LISA Pathfinder comes into play. It is a satellite that housed technology-in-testing for LISA, an ambitious project in gravitational wave physics. LISA will consist of three satellites, millions of kilometers apart from each other. Inside the satellites there will be tiny cubes, so-called test masses. Gravitational waves will induce tiny changes in the distances between the satellites (or more precisely the test masses) which will look just like a small acceleration. Exactly this effect was tested using two test masses onboard LISA Pathfinder but of course on much smaller length scales as only a single satellite was used. In principle, the setup is ideal as the space environment allows for a minimally disturbed experiment with only tiny external forces that complicate the measurement.
Unfortunately, now we come to a big issue: on the length scales of the satellite, the dark electric field looks uniform which means that both test masses experience the same acceleration. LISA Pathfinder mainly measures the relative acceleration between the test masses which means that we would not see any signal. This is a very intuitive result: Imagine you sit in a car at a red light and one traffic light ahead, a friend of yours is in the same situation. Now if both lights turn green at the same time and you both accelerate in the same way, there will be no relative motion between the two of you. Therefore, it was established in the community that LISA Pathfinder cannot be used as a direct detection experiment.
This is where our group of theorists came up with an idea. They realized that LISA Pathfinder also has very sensitive auxiliary measurements, especially one that measures the relative acceleration between the test masses and the satellite. Naively, this seems to suffer from the same problem. But we need to remember: the dark electric field couples to the number of neutrons. The test mass material (a gold-platinum alloy) has a much higher density of neutrons when compared to the rest of the satellite which induces a relative acceleration. This is shown in the accompanying figure by the different sizes of the red arrows (exaggerated for clarity) on the test masses and on the satellite.
In our car analogy, this would correspond to a third car that cannot keep up with the identical acceleration of the two cars. While you and your friend head off at a constant distance between your cars, the third car will soon fall behind. This is the simple idea behind the project that allows for the use of the LISA Pathfinder data which has been analyzed for a Dark Matter signal. These new results were able to exclude the existence of Dark Photons which are extremely light and very weakly coupled.
In unraveling the mysteries of dark matter, this collaborative effort exemplifies the interplay of theoretical insight and experimental ingenuity, while simultaneously linking two of the cornerstones of the excellence cluster Quantum Universe: Dark Matter and Gravitational Waves.