Eccentric Black Hole Binaries Could Point to a New Fundamental Particle
5 September 2024
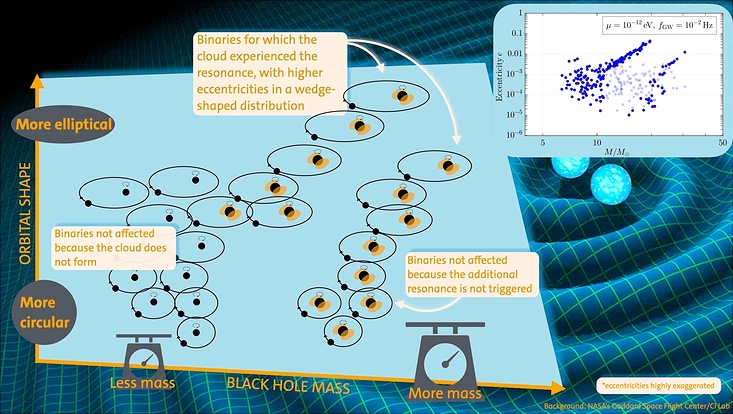
Photo: M. Koschnitzke & M. Bošković et al. (2024)
Three researchers from the Quantum Universe Excellence Cluster have found that observing black hole binaries with a higher than expected orbital eccentricity could hint at the existence of an unknown fundamental particle. Ultra-light particles, much lower in mass than any known particle, are well-motivated dark matter candidates and could produce measurable effects on gravitational-wave signals. Mateja Bošković (DESY), Matthias Koschnitzke (University of Hamburg & DESY) and Rafael Porto (DESY) have shown that such ultralight particles can also leave an imprint in the distribution of orbital eccentricities of black holes orbiting each other.
It is theoretically predicted that these particles can be produced from the rotational energy of the black hole and then accumulate in its environment in the form of a dense cloud. The researchers demonstrate that in presence of such a cloud, due to the tidal forces from a binary companion, the orbit's eccentricity – a measure of how elongated it is – can increase. The shape of the binary's orbit carries the signature that will provide tantalizing evidence for such a new fundamental particle in nature. The gravitational wave signal emitted by such a black hole binary will be readily accessible with the next generation of gravitational wave detectors like LISA and Deci-hertz.
Ultralight particles have been intensively investigated in elementary particle physics. The most prominent of these hypothetical particles is the axion, which was proposed in the 1970s to solve a problem in our understanding of the strong force that holds protons and neutrons together. Soon, it was also identified as a valid candidate for the elusive dark matter, an unidentified form of matter showing its influence only through gravitational interactions in astrophysics and cosmology. String theory, the most vital candidate for a combined description of all fundamental interactions, also supports the existence of many different light particles, usually called axion-like-particles.
For the setting of this finding, we now turn to black holes. These dense objects are characterised by their event horizon, a closed surface that cannot be crossed from the inside, not even by light. Black holes with masses a few times that of the Sun are created through the collapse of a large star that has exhausted all of its fuel and collapsed under its own gravity. Theoretically, it is possible to extract energy from a fast-rotating black hole, dubbed black hole superradiance: A wave can come close to the rotating event horizon and bounce off it in a way that it gets amplified, carrying away some of the black hole’s rotational energy. A heuristic comparison would be to think of a person jumping on a conveyor belt that is running fast. The person will be accelerated forward, with energy transferred from the belt to the person. Similarly, water waves can be amplified by wind if the wind blows faster than the waves move forward.
For light axion-like-particles, their large wavelength corresponding to their small mass allows for amplification and hence makes them perfect candidates to realise black hole superradiance. Bound to the black hole by gravity, superradiance happens in a repeated fashion, resulting in a dense cloud of particles surrounding the black hole. Since the configuration of this cloud only allows for discrete energy levels, much like the states of an electron in an atom, this object has been dubbed a gravitational atom. We point out that this would be independent of this axion-like-particle being the dark matter, it would just need to generally exist in nature.
When the gravitational atom is orbited by a second object, for example, another black hole, the orbital companion induces tidal forces, similar to those between Moon and Earth, which induce the familiar ebb and flow of water level at the Hamburg harbour. Because of the discrete energy levels of the cloud, there can be resonance effects when the orbital frequency matches the energy gap between the cloud’s energy levels. Again, this is similar to an actual atom, which can be excited only by light at particular wavelengths.
Every binary black hole spirals in closer together over time because it loses energy by the emission of gravitational waves, perturbations in space carrying away orbital energy. Because of this, the orbital frequency gradually increases, and at certain points, it can hit one of the resonance frequencies of the cloud. During such a resonant transition, the cloud is not only transformed into a state that is re-absorbed by the black hole, but it loses or gains energy and angular momentum, which is transferred to or from the orbit, respectively. It is already known that the energy transferred into the orbit during such a transition can counterbalance the loss of energy in gravitational waves, meaning that the inspiral of the two black holes stalls for some time. These are called floating transitions.
In their work, the three researchers considered, for the first time, orbits with non-zero eccentricity, which are orbits that are not circular but elliptic. Due to the more complex motion celestial objects have on elliptical orbits, the binary-cloud system allows for additional resonances at different orbital frequencies if the eccentricity is not vanishingly small. If such an additional resonance is also of the floating-type, a different interplay between energy and angular momentum transfers can now lead to the effect that, while the orbit is stalling, the eccentricity of the orbit increases, meaning that the orbit gets more elliptic.
Such a floating transition with increasing eccentricity could, for some parameters, also be observed directly by gravitational wave observatories. However, while the cloud is gone afterwards, the effect of the transition, a larger eccentricity, remains imprinted onto the orbit. During the following continued inspiral, the eccentricity naturally decreases slowly, which is why we usually expect almost circular orbits at high orbital frequency. This means observing an unusually high eccentricity at a late stage of the inspiral could be a sign that one of the black holes once carried a cloud of ultralight particles!
As a proof-of-concept, the researchers studied the history of resonances of a typical low mass population of black hole binaries formed in isolation. If there exists a single new particle, black holes with slightly different masses could all carry a cloud, while the resonance effect would happen at slightly different orbital frequencies due to the different characteristic size ratios of the particle and the black holes. Comparing all of those binaries at a particular reference frequency, the effect of the cloud and the tidal resonance could be imprinted as a peculiar feature in the distribution of eccentricities: The higher mass black holes would have experienced the resonance at a slightly higher frequency, leading to a higher remnant eccentricity at a later time. Therefore, the distribution of eccentricities in the whole population would have a skewed feature, like a wedge (see picture).
We are sure that axion-like-particles interact, at best, very weakly with ordinary matter, making them hard to detect or produce on Earth. However, it is not impossible that they interact only via gravity, which would make experimental investigation basically impossible in the foreseeable future. This research is the newest in a series of papers that try to open backdoors towards the observation of axion-like-particles. For the currently planned next generation of gravitational-wave detectors like LISA and Deci-hertz, it will be possible to observe binaries long before they merge, during their inspiral phase. Observation of binaries with higher than expected eccentricity (or even stalling frequency) could possibly hint at a new particle in nature that could be otherwise unobservable.