A New Spin on Neutron Star Mergers
18 June 2024
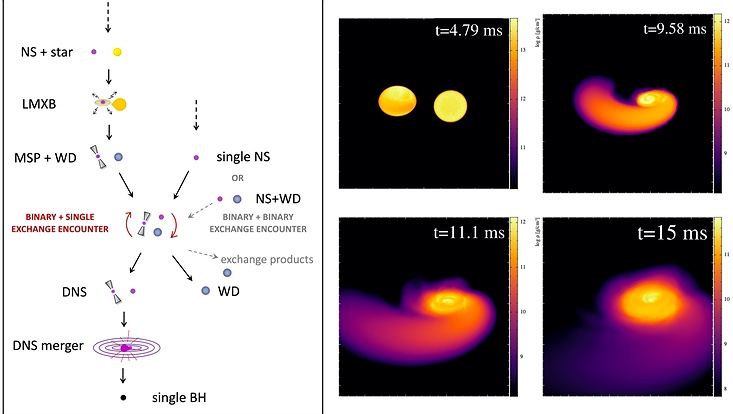
Photo: diagram and simulation by S. Rosswog et al. (2024)
Neutron star mergers are among the most violent and spectacular events in the Universe. Until now, large individual spins of the neutron stars had not been considered important, since — in the standard picture — neutron stars have a long time (~10⁸) to ‘spin down’, but hardly any time to ‘spin up’. Now a group of researchers from the Quantum Universe Excellence Cluster has realized that dense stellar clusters produce a noticeable fraction of binary systems where only one of the neutron stars is rapidly spinning. Using innovative numerical relativity methods, they find that such mergers are weaker gravitational wave emitters, but produce particularly bright and red electromagnetic emission known as ‘kilonova’.
Neutron stars are the collapsed cores of massive stars measuring roughly 10 km in radius yet wholly composed of extremely dense material - mostly neutrons - at nuclear densities. A sugar cube of neutron star material would weigh more than a large mountain on Earth.
A merger between two neutron stars occurs only rarely within a galaxy, roughly once every 100 000 years. Nevertheless, such events are related to many important questions in fundamental physics and astrophysics. The orbital motion of the first observed neutron star binary (Hulse-Taylor pulsar) has indirectly, but definitely, proven that gravitational waves actually do exist and are not merely a mathematical artefact. This settled a long debate about the existence of gravitational waves. The measurement of relativistic orbital effects also allows for an extremely accurate mass measurement (the masses of the Hulse-Taylor pulsar binary are known to within 1 part in 105) and such accurately determined masses have been crucial in constraining the properties of nuclear matter beyond the densities in atomic nuclei.
Moreover, such neutron star mergers cause rapid changes in strongly curved spacetime due to their gravity and therefore are strong emitters of gravitational waves. They can be used to determine the Hubble parameter and their presence tells us a lot about the evolution of binary stars. As the authors had already predicted in the late 90s, such mergers eject neutron rich matter into space which forges a good fraction of the heaviest nuclei and therefore contributes in the enrichment of the cosmos with heavy elements. These freshly synthesized and initially radioactive elements cause electromagnetic flashes (so-called kilonovae) that are tell-tale observational signatures of the ongoing nucleosynthesis. Last, but not least, the merger of two neutron stars was for a long time suspected to cause bright cosmic explosions called gamma-ray bursts. All of these, originally purely theoretical, ideas were splendidly confirmed by the first direct detection of a neutron star merger (GW170817) in August 2017.
The researchers used their newly developed Lagrangian numerical relativity code SPHINCS_BSSN to simulate neutron star mergers where one of the neutron stars had a fast spin (~1 millisecond period) while the other had no spin. They found several distinct signatures which will be relevant to that fraction of all NS mergers which contain one high-spin component, expected most in globular clusters. The simulations show only one very pronounced tidal tail is produced upon merger. This is similar to the case of unequal mass mergers, since spinning neutron stars are strongly rotationally flattened and therefore more susceptible to tidal effects. Ten times more unshocked material is found to be ejected out of the merger producing a substantially brighter and redder burst of electromagnetic radiation known as the kilonova.
The spinning case mergers are overall less violent and eject smaller amounts of shock-generated semi-relativistic material. This implies weaker blue or ultraviolet precursor signals to the kilonava. They also see more accreting material falling back which will power late-time X-ray flares. Importantly, the post-merger remnant is seen to power gravitational waves less efficiently. This could delay a potential collapse to a black hole in such high-spin neutron star mergers.
Future gravitational wave and electromagnetic observations of binary neutron star mergers will have to carefully watch out for the signatures of this sub-population of spinning binary mergers.
What Do We Learn from Neutron Star Collisions?
Further Information on this subject and on the ERC Advanced Grant project “INSPIRATION: From inspiral to kilonova”:
Where in the cosmos were the heaviest elements forged? What are the densest forms of matter in the Universe? What happens when two neutron stars collide? What do their observable signals tell us about so-far incompletely understood physics?